Development and validation of an HPTLC-DPPH assay method for the acteoside content of Ribwort ipowder®
The French company PiLeJe Industrie develops liquid and dry plant-derived ingredients using patented procedures and in-house developed processes. Their products are mainly used in the food supplements industry. The long-term collaboration with Chromacim led to the important development of HPTLC in their laboratory. This article addresses the question, whether it is possible to validate the activity assay of one major compound through its activity only, using the best international current standard.
Introduction
Ribwort plantain (Plantago lanceolata L.) is a common grassland plant traditionally used for its therapeutic properties. The leaves are used in many European countries for the symptomatic treatment of colds and inflammation of the mouth and throat. Biological activities of P. lanceolata include antihistaminic, anti-spasmodic, anti-nociceptive, neuroprotective, metabolic, and gastro-protective activities. Acteoside is a phenyl-propanoid glycoside, well known for its antioxidant and antiinflammatory properties, which is commonly used as a marker.
Ribwort ipowder® is a plant infusion concentrated on plant totum, a proprietary 100% plant-based product developed by PiLeJe Industrie, made from dried P. lanceolata according to a patented process [1, 2]. Quantification was already shown with standard detection and published in a previous issue of the CBS. Our goal was to prove that this type of activity detection is reliable and transferable to quality assurance with a proper validation package. This needs a level of knowledge that we have developed rather quickly with the help of our partners. The objectives of this work were 1) to develop an HPTLC method using the 2,2-diphenyl 1-picrylhydrazyle (DPPH*) effect-directed chemical reaction for the detection of the antioxidant activity of acteoside for quality control of industrial dry extracts of P. lanceolata and 2) to demonstrate the applicability of the concept of Life Cycle Management of analytical methods to quantitative HPTLC-DPPH* methods.
Standard solution
An acteoside standard is dissolved in methanol at a concentration of 17.40 μg/mL in methanol.
Sample preparation
500 mg of Ribwort ipowder® (PiLeJe Industrie) are extracted with 40 mL of ethanol – water 50:50 (V/V) by sonication at 60 °C for 10 min, then filtered and transferred to a 50 mL volumetric flask and filled up to the mark. The solution is diluted 5-fold for application.
Chromatogram layer
HPTLC plates silica gel 60, 20 x 10 cm are used.
Sample application
4.0 μL of sample and standard solutions are applied as bands with the Automatic TLC Sampler (ATS 4), 20 tracks, band length 8.0 mm, distance from left edge 22.0 mm, distance from lower edge 8.0 mm.
Chromatography
Plates are developed with ethyl acetate – water – acetic acid – formic acid 100:27:11:11 (V/V) to 70 mm (from the lower edge) in the ADC 2 with chamber saturation (20 min, with filter paper) and after activation at 33 % relative humidity for 10 min using a saturated aqueous solution of magnesium chloride.
Post-chromatographic derivatization
After drying for 10 min, the plates are immersed into DPPH* reagent (0.5 mM methanolic solution of 2,2-diphenyl-1-picrylhydrazyl, immersion speed 5 cm/s, immersion time 5 s) with the Chromatogram Immersion Device 3. The plates are dried at room temperature in the dark for 90 s and then heated at 60 °C for 30 s (TLC Plate Heater 3).
Documentation
Images of the plates are captured with the TLC Visualizer in white light after derivatization.
Densitometry
Fluorescence mode is used for measurement at 517 nm (tungsten lamp) with TLC Scanner 4 and visionCATS to obtain a positive response of the peaks of interest.
Results and discussion
Analytical methods used for quality control of plants and plant extracts are usually based on the identification and quantification of chemical markers to manage batch reproducibility and efficacy. To measure the concentration of acteoside in Ribwort ipowder®, the HPTLC DDPH* assay was applied. The assay determines the free radical scavenging activity of the plant extract in solution.

Figure 1: Antioxidative properties – DPPH
The first step of this work was the selection of the Analytical Target Profile (ATP) and the determination of the Target Measurement Uncertainty (TMU) taking into account the quality control requirements for such extracts and the applicable range of the detection method. Once the desired range was established, an evaluation of the calibration function was conducted using linear, 1/x and 1/x2 weighted linear calibration models and those three models were used to assess accuracy of the method (trueness and precision) by means of accuracy profiles [3]. The 1/x2 weighted linear calibration function showed the best performance in the tested range, both in terms of accuracy and uncertainty of measurement.
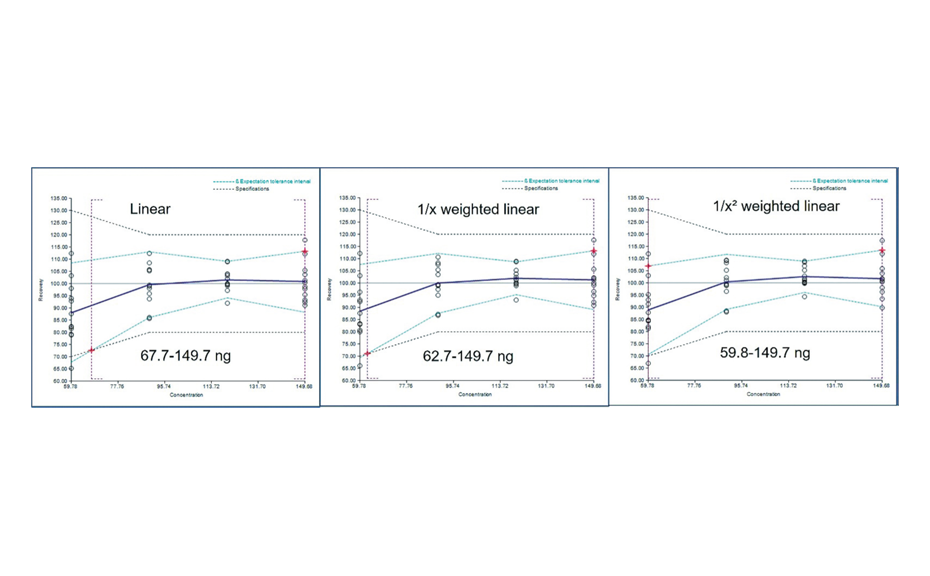
Figure 2: Accuracy profiles obtained with the selected calibration function, for each function the accuracy limits are given by the vertical doted lines
The method requirement was to assay acteoside amounts around 1.0–2.0% (W/W) in industrial dry extracts of Ribwort plantain with an acceptance criterion of ± 20.0% difference to the true value for the ATP (defined as the combination of the trueness (bias) and precision characteristics), and a TMU of less than 20.0–25.0% relative uncertainty, according to the quality control needs.
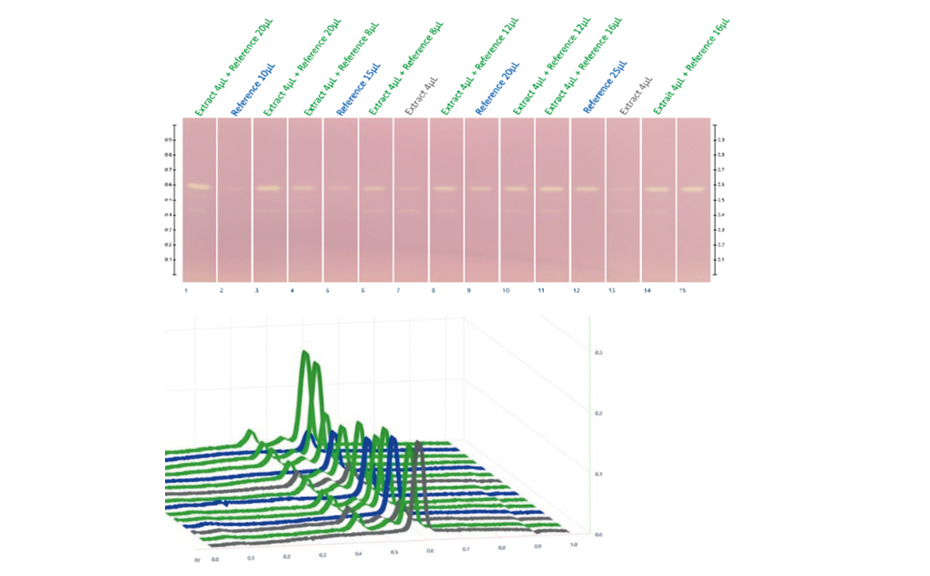
Figure 3: HPTLC chromatogram in white light and densitograms measured in fluorescence mode at 517 nm with a tungsten lamp after DPPH* assay of standards and plantain leaf extract
Due to the lack of reference samples, spiked samples were used to evaluate the accuracy of the method by means of Total Analytical Error (TAE) determination, using prediction intervals calculation for the selected calibration functions. For quality control, the calibration function with the best performance level in accordance with the product specifications was chosen by estimating the Measurement Uncertainty (MU).
As Life Cycle Management of the method also includes its routine use, the MU was checked on spiked and non-spiked extract samples at different dilution levels, in order to verify the accordance of results between those samples, and to prepare a replication strategy for the routine method. Statistical calculations were performed with NeoLiCy® software for analytical methods’ life cycle statistical assessment (NeoLiCy, Marseille- Mâcon, France). The tested dilutions did not show any significant effect on the calculated spiked amount and any significant impact on the extract calculated concentrations. To take care of the measurement dispersion we included repeated sample preparation and measurement in the analytical procedure.
This work demonstrated that the concept of Life Cycle Management of analytical methods can successfully be applied to a HPTLC-DPPH* method, even in the case of complex matrices such as plant extracts, from the definition of the ATP and TMU to the reflection on the replication strategy to be applied in quality control.
The method developed for the quantification of acteoside in Ribwort plantain is applicable in a working range from 75.0 to 225.0 ng of acteoside and fit for purpose for use in quality control laboratories.
This study showed the suitability of HPTLC in this domain. Furthermore, the partnership with Chromacim and NéoLiCy showed its efficiency, within a cumulative working time of less than two weeks only, including statistics and matrix effect evaluation. This method is therefore ready to be transferred to the quality control laboratory of the PiLeJe Group.
This success proves that we were initially right to select this approach and encourages us to continue in this powerful way to develop HPTLC as a relevant technique for our needs.
Literature
[1] Dubourdeaux, M. Procédé de Préparation d’extraits Végétaux Permettant l’obtention d’une Nouvelle Forme Galénique. 14 January 2009. Available at https://patents.google.com/patent/EP2080436A2/en, accessed on January 21, 2021)
[2] V. Bardot et al. (2020) Food Funct, https://doi.org/10.1039/c9fo01144g
[3] J.M. Roussel et al. (2021) J Chromtogr B, https://doi.org/10.1016/j.jchromb.2021.122923
Contact: Dr. Valérie Bardot, Naturopôle Nutrition Santé, Les Tiolans, 03800 Saint-Bonnet de Rochefort, France, v.bardot@pileje.com